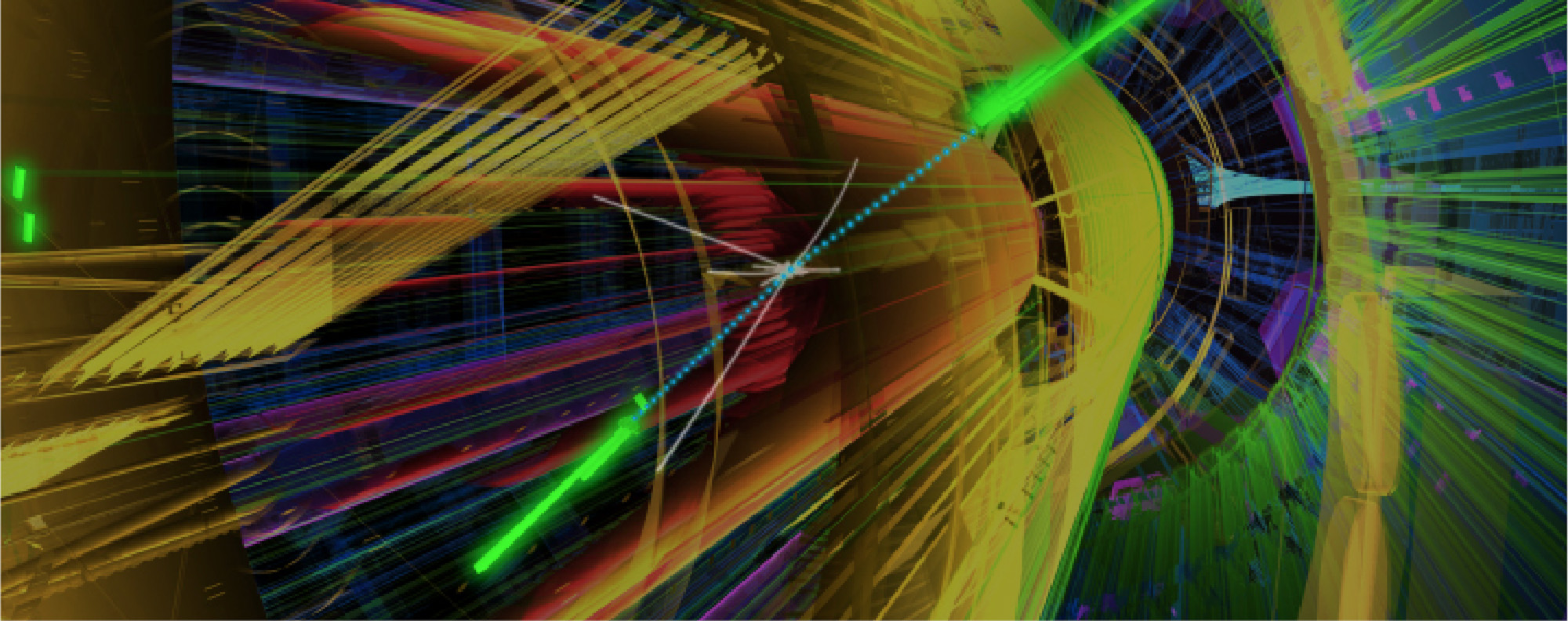
The LHC will operate at 13 TeV – nearly double its previous energy – opening new frontiers in our understanding of the fundamental structure of matter
In early 2013, the Large Hadron Collider (LHC) shut down for two years of planned maintenance and repairs. Now the physics programme at the world’s most powerful accelerator is ready to restart, this time at 13 TeV — almost double its previous energy. This new energy frontier will allow researchers to probe new boundaries in our understanding of the fundamental structure of matter.
The Higgs boson
On 4 July 2012, the ATLAS and CMS experiments at CERN announced the discovery of a Higgs boson, a particle with a mass of 126 GeV. The Higgs is the simplest manifestation of the Brout-Englert-Higgs mechanism that gives particles mass. It is the final particle in the Standard Model – the theory that explains the fundamental particles and the forces between them – to be experimentally verified. Increasing the energy of the LHC will increase the chance of creating Higgs bosons in collisions, which means more opportunity for researchers to measure the Higgs precisely and to probe its rarer decays. High-energy collisions could detect small, subtle differences between what the boson looks like in experiments, and what the Standard Model predicts.
Exotic particles
Some theories predict that there could be a whole new set of particles out there that physicists cannot detect because they don’t interact through the electromagnetic force. But if these “dark sector” particles have mass, they will interact with the field associated with the Higgs boson. So the Higgs boson becomes a contact point between the Standard Model and new, more exotic particles.
Dark matter
Invisible dark matter makes up most of the universe – but we can only detect it from its gravitational effects. But what is dark matter? One idea is that it could contain “supersymmetric particles” – hypothesized particles that are partners to those already known in the Standard Model. The data from higher-energy running at the LHC could provide more direct clues to resolve this mystery.
Supersymmetry
The Standard Model has worked beautifully to predict what experiments have shown so far about the basic building blocks of matter, but the theory is incomplete. Supersymmetry is an extension of the Standard Model that aims to fill some of the gaps. It predicts a partner particle for each particle in the Standard Model. These new particles would solve a major problem, fixing the mass of the Higgs boson. If the theory is correct, supersymmetric particles should appear in high-energy collisions at the LHC.
Extra dimensions
Why is gravity so much weaker than the other fundamental forces? Perhaps we don’t feel the full effect of gravity because part of it spreads to extra dimensions. But how could we test for extra dimensions? One option is to find evidence of particles that can exist only if extra dimensions are real. Theories that require extra dimensions predict that, just as atoms have a low-energy ground state and excited high-energy states, there would be heavier versions of standard particles in other dimensions. Such heavy particles could be revealed at the high energies the LHC will reach in Run 2.
Antimatter
Every particle of matter has a corresponding antiparticle, exactly matching the particle but with opposite charge. For the electron, for example, there is an “antielectron” called the positron – identical in every way but with a positive electric charge. But when matter and antimatter come into contact, they annihilate, disappearing in a flash of energy. The Big Bang should have created equal amounts of matter and antimatter. So why is there far more matter than antimatter in the universe? Running at higher energy will allow the production of more antiparticles for CERN’s antimatter programme – helping physicists to check if the properties of antimatter differ from those of matter.
Quark–gluon plasma
For a few millionths of a second, shortly after the Big Bang, the universe was filled with an astonishingly hot, dense soup made of all kinds of particles moving at near light speed. This mixture was dominated by quarks – fundamental bits of matter – and by gluons, carriers of the strong force that normally “glue” quarks together into familiar protons, neutrons and other species. In those first evanescent moments of extreme temperature, however, quarks and gluons were bound only weakly, free to move on their own in what’s called a quark–gluon plasma. The higher energy collisions at the LHC will allow new and more detailed characterization of this quark-gluon plasma.
Downloads
Attachment | Size |
---|---|
![]() | 749.69 KB |